SupplementsAugust 10, 2012 / 61(04);1-9
Mary Jean Brown, ScD
Stephen Margolis, PhD
Division of Emergency and Environmental Health Services, National Center for Environmental Health
Corresponding author: Mary Jean Brown, ScD, National Center for Environmental Health, CDC, 4770 Buford Highway NE, MS F-60, Atlanta, GA 30084. Telephone: 770-488-3300; Fax: 770-488-3635; E-mail: mjb5@cdc.gov.
Introduction
Lead is a pervasive environmental contaminant. The adverse health effects of lead exposure in children and adults are well documented, and no safe blood lead threshold in children has been identified. Lead can be ingested from various sources, including lead paint and house dust contaminated by lead paint, as well as soil, drinking water, and food. The concentration of lead, total amount of lead consumed, and duration of lead exposure influence the severity of health effects. Because lead accumulates in the body, all sources of lead should be controlled or eliminated to prevent childhood lead poisoning. Beginning in the 1970s, lead concentrations in air, tap water, food, dust, and soil began to be substantially reduced, resulting in significantly reduced blood lead levels (BLLs) in children throughout the United States. However, children are still being exposed to lead, and many of these children live in housing built before the 1978 ban on lead-based residential paint. These homes might contain lead paint hazards, as well as drinking water service lines made from lead, lead solder, or plumbing materials that contain lead. Adequate corrosion control reduces the leaching of lead plumbing components or solder into drinking water. The majority of public water utilities are in compliance with the Safe Drinking Water Act Lead and Copper Rule (LCR) of 1991. However, some children are still exposed to lead in drinking water. EPA is reviewing LCR, and additional changes to the rule are expected that will further protect public health. Childhood lead poisoning prevention programs should be made aware of the results of local public water system lead monitoring measurement under LCR and consider drinking water as a potential cause of increased BLLs, especially when other sources of lead exposure are not identified.
This review describes a selection of peer-reviewed publications on childhood lead poisoning, sources of lead exposure for adults and children, particularly children aged <6 years, and LCR. What is known and unknown about tap water as a source of lead exposure is summarized, and ways that children might be exposed to lead in drinking water are identified. This report does not provide a comprehensive review of the current scientific literature but builds on other comprehensive reviews, including the Toxicological Profile for Lead and the 2005 CDC statement Preventing Lead Poisoning Among Young Children (1,2). When investigating cases of children with BLLs at or above the reference value established as the 97.5 percentile of the distribution of BLLs in U.S. children aged 1–5 years, drinking water should be considered as a source. The recent recommendations from the CDC Advisory Committee on Childhood Lead Poisoning Prevention to reduce or eliminate lead sources for children before they are exposed underscore the need to reduce lead concentrations in drinking water as much as possible (3).
Background
Lead is a relatively corrosion-resistant, dense, ductile, and malleable metal that has been used by humans for at least 5,000 years. During this time, lead production has increased from an estimated 10 tons per year to 1,000,000 tons per year, accompanying population and economic growth (4). The estimated average BLL for Native Americans before European settlement in the Americas was calculated as 0.016 µg/dL (5). During 1999–2004, the estimated average BLL was 1.9 µg/dL for the noninstitutionalized population aged 1–5 years in the United States (6), approximately 100 times higher than ancient background levels, indicating that substantial sources of lead exposure exist in the environment.
BLLs of U.S. children increased sharply during 1900–1975 as increased lead use and emissions caused widespread environmental contamination across the United States. Changes in federal laws to limit the use and emissions of lead have reversed this trend. Effective regulations include reducing or eliminating lead from gasoline for on-road vehicles, foods and food packaging, house paint, water pipes, plumbing fixtures, and solder used in plumbing and drink cans.
Effects of Lead Exposure
Effects on Children
The health consequences of lead exposure depend on the cumulative dose of lead and vulnerability of the individual person rather than the environmental media (i.e., food, water, soil, dust, or air) in which the lead exists. Compelling evidence has established the cognitive effects of childhood lead exposure since they were first described in 1943 (7). To date, no safe blood lead threshold for the adverse effects of lead on infant or child neurodevelopment has been identified (2). Recent evidence suggests that the dose-effect relationship might be supralinear, with a steeper dose response and potential risk for an adverse health effect such as IQ loss at BLLs <10 µg/dL compared with BLLs ≥10 µg/dL (8–10). The developing fetus and child are more sensitive to lead exposure than adults because of the immaturity of the blood-brain barrier, increased gastrointestinal absorption, and hand-to-mouth behaviors, all of which increase exposure (11). Comorbidities such as iron deficiency also can enhance lead absorption.
Evidence from several prospective studies suggests that the adverse effects of early childhood exposure on neurodevelopment persist into the second decade of life (12–16). The mechanisms by which low levels of lead exposure might adversely affect neurobehavioral development remain uncertain, although experimental data support the involvement of many physiological pathways.
Effects on Adults
Overall. Adults with occupational exposure to lead report more colds and influenza and exhibit suppressed secretory immunoglobulin A (IgA) levels, demonstrating lead-induced suppression of humoral immunity (17). Adults with occupational exposure also might have neurotoxic effects, including peripheral neuropathy. Motor nerve dysfunction can occur at BLLs as low as 40 µg/dL (18). Lead also is nephrotoxic and can cause progressive nephron loss leading to renal failure, gout, and hypertension. In a meta-analysis of the relationship between BLL and blood pressure, a small but statistically significant association between increased BLL and increased blood pressure was identified (19). BLLs ≥40 µg/dL have been associated with increased risk for cardiovascular, cancer, and all-cause mortality in several epidemiological studies. These effects might not be limited to adults with the long-term, high-dose exposure common in occupational settings. A study of approximately 13,000 adult participants in the third National Health and Nutrition Examination Survey (NHANES) with 12 years of follow-up found that adults in the highest tertile of BLL (≥3.6 µg/dL) were at increased risk for all-cause mortality and cardiovascular mortality but not for cancer mortality compared with those in the lowest tertile of BLL (<1.9 µg/dL) (20).
Reproductive and Prenatal Effects. Lead exposure remains a concern for pregnant and lactating women, particularly those who have an occupational exposure to lead, who are recent immigrants, who are engaged in home renovations, or who have pica. Prenatal lead exposure resulting in maternal BLLs <10 µg/dL has measurable adverse effects on maternal and infant health, such as fertility, hypertension, and infant neurodevelopment (21). In addition, because lead persists in bone for decades, as bone stores are mobilized to meet the increased calcium needs of pregnancy and lactation, women and their infants might be exposed to lead long after external sources have been removed (22). Adverse reproductive effects are not limited to women. In males with occupational lead exposure, abnormal sperm morphology and decreased sperm count have been observed at BLLs <40 µg/dL (23).
Carcinogenic Effects
Based on limited evidence from studies in humans and sufficient evidence from animal studies, the U.S. Department of Health and Human Services (HHS) has determined that lead and lead compounds are reasonably anticipated to be human carcinogens, and the U.S. Environmental Protection Agency (EPA) has determined that lead is a probable human carcinogen. The International Agency for Research on Cancer also has determined that inorganic lead is likely carcinogenic in humans (1).
Scope of Public Health Concern
In 2004, 143,000 deaths and a loss of 8,977,000 disease-adjusted life years were attributed to lead exposure worldwide, primarily from lead-associated adult cardiovascular disease and mild intellectual disability in children (24). Children represent approximately 80% of the disease impact attributed to lead, with an estimated 600,000 new cases of childhood intellectual disabilities resulting from BLLs ≥10 µg/dL each year (25).
In 1987, one study estimated that reducing water lead levels to below the maximum contaminant level for lead (which was 20 parts per billion [ppb] during 1986–1991) would save nearly $400 million per year (1985 dollars) (26). Although estimates of the reductions in lead concentrations for various media, air, dust, soil, and water are unavailable, reductions in BLLs were observed for each subsequent cohort of children aged 2 years who were not exposed to lead at the concentrations experienced by the cohort of children aged 2 years in 1976. As a result of the overall reduction of lead in the environment, including fuel, house paint, and drinking water, there was an estimated decrease in BLLs of 15.1 µg/dL and a related estimated economic benefit of $110–$300 million in earnings for children born after 1976 who were not exposed to high levels of lead (27). A recent cost-benefit analysis suggested that for every dollar spent to reduce lead hazards, $17–$220 is saved. This cost-benefit ratio compares favorably to that of other public health interventions such as vaccines (28).
Historical Trends in Blood Lead Levels
Since the 1970s, NHANES data have been used to track BLLs for the noninstitutionalized U.S. population (Figure). In 1978, approximately 13.5 million children aged 1–5 years had BLLs ≥10 µg/dL, which is generally considered the threshold for housing inspection, health education, and home visits (i.e., case management) by local and state public health agencies. By 2007–2008, this number had decreased to approximately 250,000 (29). In addition, in the early NHANES data from the 1970s and 1980s, 12% of black children had BLLs ≥30 µg/dL, compared with 2% of their counterparts who were white. Children living in low-income families also were at greater risk for BLLs ≥30 µg/dL (10% of children in households earning <$6,000 per year compared with 1.2% living in households earning >$15,000 per year) (30). By the 1999–2004 surveys, the percent differences in BLLs ≥10 µg/dL for children aged 1–5 years, by race and family income, were no longer statistically significant, a trend that continues today at levels ≥10 µg/dL, demonstrating the impact of policies at the federal, state, and local levels (6).
In the 1991–1994 NHANES, the overall prevalence of BLLs ≥10 µg/dL was 2.2% but decreased to 0.7% by the 1999–2002 survey. Overall, the geometric mean (GM) decreased significantly (p<0.05; two-tailed t-test) from 2.3 µg/dL to 1.6 µg/dL during the same time period. Among children aged 6–12 years, boys had significantly higher GM BLLs than girls in all age, racial, and income groups (6).
Despite the considerable progress in decreasing BLLs, children aged <6 years continue to be exposed to lead. Although disparities among various subpopulations of children with BLLs ≥10 µg/dL are no longer significant, disparities in risk for exposure have persisted over time. In addition, mean BLLs continue to be higher for children from low-income families, non-Hispanic black children, and children living in older housing (i.e., built before 1950) (6). For example, the mean BLL for non-Hispanic black children (1.9 µg/dL) was significantly higher (36%) than that of white children (1.4 µg/dL) during 2007–2008.
Although the decrease in GM BLLs in women aged 20–59 years from 1.8 µg/dL in 1988–1994 to 1.2 in 1999–2002 was similar to that seen in children, in utero exposure is also a substantial public health issue for certain populations, particularly new immigrants (31–33). In a 2003 study conducted in New York City, New York, BLLs ≥5 µg/dL were more prevalent among pregnant women who were born outside the United States than pregnant women born in the United States (odds ratio = 8.2, 95% confidence interval = 3.8–17.3) (34).
Lead in the Environments of Children
A review of the sources of lead in the environments of U.S. children discusses the contributions of various lead-contaminated media to BLLs in children (35). Deteriorating lead paint and lead in house dust and soil are the primary, and often the most concentrated, sources of lead. However, lead paint contamination is not the only source of exposure for U.S. children.
Lead is used in thousands of applications, each constituting a potential exposure source (36). Case reports from local and state lead programs indicate that up to 30% of children with BLLs ≥10 µg/dL do not have an immediate lead paint hazard. For example, in 2004 in Arizona, lead-contaminated soil was the most commonly identified proximate exposure source, accounting for approximately 24% of increased BLLs in children, followed by paint (17%), folk remedies and pottery (17%), dust (15%), and miscellaneous other sources (19%). In 8% of cases, no lead hazard was identified (37).
In field investigations, nonpaint lead exposure sources might be insufficiently characterized and their importance underestimated. Lead program inspectors look for lead paint hazards in places where children with BLLs ≥10 µg/dL spend time. Often, lead exposure sources other than paint are sought only when no lead paint hazards are found. Of 35 CDC-funded childhood lead poisoning prevention programs, only 15 reported that they routinely tested lead levels in water in homes where children have increased BLLs (CDC, unpublished data, 2009). Thus, for some children with BLLs ≥10 µg/dL, important nonpaint sources such as water might not be identified.
Evidence also suggests that for children with BLLs 5–9 µg/dL, no single source of exposure predominates. For these children, the contribution of multiple sources, including drinking water, seems likely, particularly for children who do not have well-established risk factors such as living in old housing or having a parent who is exposed to lead at work (38). CDC and its Advisory Committee on Childhood Lead Poisoning Prevention concur that primary prevention of lead exposure is essential to reducing high BLLs in children and that reducing water lead levels is an important step in achieving this goal.
Lead in Drinking Water
Lead is unlikely to be present in source water unless a specific source of contamination exists. However, lead has long been used in the plumbing materials and solder that are in contact with drinking water as it is transported from its source into homes. Lead leaches into tap water through the corrosion of plumbing materials that contain lead (26,39). The greater the concentration of lead in drinking water and the greater amount of lead-contaminated drinking water consumed, the greater the exposure to lead. In children, lead in drinking water has been associated both with BLLs ≥10 µg/dL (40,41) as well as levels that are higher than the U.S. GM level for children (1.4 µg/dL) but are <10 µg/dL (42–44)
History of Studies on Lead in Water
In 1793, the Duke of Württemberg, Germany, warned against the use of lead in drinking water pipes, and in 1878, lead pipes were outlawed in the area as a result of concerns about the adverse health effects of lead in water (45). In the United States, the adverse health consequences of lead-contaminated water were recognized as early as 1845 (46). A survey conducted in 1924 in the United States indicated that lead service lines were more prevalent in New England, the Midwest, Montana, New York, Oklahoma, and Texas (47). A nationwide survey conducted in 1990 indicated that 3.3 million lead service lines were in use, and the areas where they were most likely to be used were, again, the midwestern and northeastern regions of the United States. This survey also estimated that approximately 61,000 lead service lines had been removed through voluntary programs during the previous 10 years (48).
Research on exposure to lead in water increased as concern about the topic increased, and efforts were made to establish a level of lead in water that, at the time of the studies, was considered acceptable. A 1972 study in Edinburgh, Scotland, obtained 949 first-flush water samples (i.e., samples of water from the tap that have been standing in the plumbing pipes for at least 6 hours) matched with 949 BLLs, as well as 205 running water samples matched to 205 BLLs (49). No dose-response relationship could be determined when comparing BLLs with four levels of lead in both first-flush water and in running water (<0.24 µmol/L; 0.24–0.47 µmol/L; 0.48–1.43 µmol/l; and ≥1.44 µmol/L). The study concluded that the findings challenged whether it was necessary to lower the water lead concentration to <100 ppb, which at that time was the acceptable concentration established by the World Health Organization. However, the study also reported that low levels of environmental lead exposure could have adverse health effects; therefore, knowing the degree of lead exposure from household water relative to other sources is important. Another study, in 1976, of 129 randomly selected homes in Caernarvonshire, England, reported a similar finding (50), describing the relationship between blood and water lead as slight.
Comparing studies on the relationship between BLLs and lead levels in water are difficult given that the age of study participants, water sample collection methods, and duration of the exposure to high water lead levels vary considerably across studies. Quantifying the contribution of lead in water to BLLs in children can be particularly challenging because of the difficulty of collecting valid, reliable, and reproducible water lead samples. Relatively small fluctuations in factors such as temperature, pH alkalinity, and dissolved solids affect the solubility of lead (51). In addition, intake estimation is difficult because of the incomplete understanding of individual children’s water intake patterns. Nonetheless, during the 1900s, an association between blood and water lead levels continued to be reported, particularly in sensitive populations such as pregnant women and children. For example, in the Glasgow Duplicate Diet Study, the correlation coefficient between infant BLLs at age 3 months and composite water samples was 0.59 (52). In a study of the association between children’s BLLs and water lead levels, with a convenience sample of 320 households, water lead levels explained as much as 12% of the variance in BLLs (53). BLLs also were shown to decrease when lead lines were completely replaced. In one study comparing water lead levels in housing of low-income persons before and after lead service lines were replaced, water lead levels decreased by 50% in the 3 months after total replacement of lead pipes from the distribution systems (54).
In the 1983 British Regional Heart Study, the BLLs of 7,378 men in 24 British towns were evaluated (55). All men were categorized based on lead concentration in domestic water, water hardness, and alcohol and cigarette consumption. The findings indicated that when first-draw home tap water measured 100 ppb, BLLs of the men were 1.00 µmol/L, compared with 0.7 µmol/L in men with home tap water that contained undetectable lead levels. The study recommended that lead in water be given greater priority than in the past in any national campaign to reduce lead exposure. In a follow-up study in Glasgow, Scotland, tap water lead concentrations and maternal BLLs from 1981 were compared with water and BLLs from 1993 (56). In 1993, 17% of 1,812 homes had daytime, first-draw lead levels in 1 L of water that were ≥10 ppb, compared with 49% of 131 homes enrolled in a survey in 1981. Maternal BLLs decreased by 31% during the same period (11.9 µg/dL in 1981 vs. 3.7 µg/dL in 1993). Despite this decrease, the study reported that even the reduced levels of lead in tap water might have presented a risk for bottle-fed infants.
The lead studies discussed above exclusively assessed lead in water, and tap water was the only source considered as the cause of the increased BLL. One landmark U.S. observational study conducted during the 1990s studied 183 urban children aged 12–31 months (37%, 12–18 months; 31%, 18–24 months; 33%, 24–31 months) (57). Of these children, 63% had BLLs <10 µg/dL, 20% had BLLs of 10–14 µg/dL, 8% had BLLs of 15–19 µg/dL, and 3% had BLLs ≥20 µg/dL. The study evaluated lead in home foundation perimeter soils, potable water, paint, and house dust. Lead-contaminated house dust was the major contributor to BLLs ≥10 µg/dL. However, the study reported that although lead-contaminated water had a statistically significant effect on children’s BLLs after adjusting for other sources of lead exposure, no statistically significant contribution for drinking water could be discerned at levels below the current EPA action level of 15 ppb (57). Lead dust as a primary source of lead for children with increased BLLs was further demonstrated in a subsequent pooled analysis of 12 studies; floor dust lead levels as low as 5 µg/ft2 were associated with 5% of enrolled children having a BLL ≥10 µg/dL (58).
LCR and Control Measures
In 1991, EPA promulgated LCR. LCR sets an action level of 15 ppb of lead in 1 L of first-draw water taken after the water has been standing in the pipes for at least 6 hours. LCR also establishes a nonenforceable maximum contaminant level goal (MCLG) of 0, the level of lead in drinking water at which no adverse health effects are likely to occur. However, EPA has determined that MCLG is not feasible because many sources of lead in water are not under the control of public drinking water suppliers (i.e., water utilities). LCR requires water utilities to monitor lead in drinking water from a sample of customer taps in homes with plumbing materials that contain lead and copper. If >10% of the samples collected from a water utility serving <50,000 residents exceeds the lead action level, the utility must identify and install optimal corrosion control treatment. Utilities serving ≥50,000 residents are required to have optimal corrosion control treatment regardless of level of lead in drinking water. Any size water utility exceeding the lead action level and covered by LCR is required to educate the public about lead in drinking water until water levels are below the lead action level. Utilities that exceed the action level must distribute public education materials on lead to customers and organizations that serve consumers with populations at high risk for adverse health effects from lead (e.g., schools, pediatricians, and child-care centers). The public education materials must include information about the health effects of lead, sources of lead, and steps persons can take to reduce their exposure to lead.
LCR requires that additional action be taken when a water utility with lead service lines and optimized corrosion control treatment still exceeds the action level in >10% of samples collected. In addition to public education, these utility companies also are responsible for replacing the portion of the lead service lines (the line connecting a house to the water distribution system) that the utility company owns. Utility companies must offer to replace the section of line owned by the customer (the section from the water meter into the house). However, the utility company is not required to bear the cost of replacing the privately owned portion of the line. When a customer does not agree to replacement of the privately owned portion of the line, the utility company must notify the residents at least 45 days in advance that they might experience a temporary increase in water lead levels as the portion of the service line owned by the utility company is replaced and must provide guidance on measures to minimize their exposure to lead. A utility company also must collect a water sample within 72 hours after completion of the partial replacement, test the sample for lead, and notify the customer of the results.
Most U.S. drinking water systems are in compliance with LCR. In 2004, the Congressional Research Service reported that an EPA review of its water monitoring data conducted during 2000–2003 did not find a systemic problem of increased lead levels among water systems. For systems that serve >50,000 persons, 27 (3.6%) of these systems exceeded the action level of 15 ppb at least once in the time period. For systems that served 3,300–50,000 people, 237 (3.4%) exceeded the action level at least once since 2000 (59). However, lead service lines remain in use in neighborhoods in many cities. These pipes range in age from those installed during the late 1800s through 1986, although the preponderance of lead service line installations occurred before World War II.
Changes in water treatment and disinfection practices can substantially undermine lead corrosion control (60). In the mid-1990s in the District of Columbia (DC), high levels of free chlorine were used to decrease coliform bacteria in water, a process that inadvertently changed the type of lead mineral coating in the water lines to one with very low solubility in the background pH of the DC drinking water. When the free chlorine was replaced with chloramines, the transformed highly insoluble lead scale minerals were no longer stable and dissolved. Therefore, a substantial level of lead was released from the lead service lines into drinking water at the tap (61). CDC reviewed the relationship between BLLs in children, the presence of a lead service line, and water disinfection practices in DC during 1998–2006 (62). The study reported that the presence of a lead service line was associated with higher BLLs in children. This relationship was most pronounced during 2001 through June 2004, when chloramines were used to disinfect the drinking water without adequate corrosion control (62). An observational study in which the BLLs of children were matched to population-based data of water lead levels during periods when water disinfection practices changed in DC concluded that the increase in water lead levels was associated with an increase in the BLLs of children (63). In a study in Wayne County, North Carolina, a unified geographic information system was used to link BLLs with water service lines made with or without lead. The use of chloramines in water predicted higher water lead levels and BLLs, controlling for well-established, known predictors of BLL (44).
Partial replacement of lead service lines might not effectively decrease the increased BLLs associated with lead service lines, as shown by one observational study in DC during July 2004–December 2006 that assessed the BLLs and type of water service line (62). Compared with children who had never had a lead service line, children having had a partial lead pipe replacement were at increased risk for increased BLLs, and BLLs of children with partial lead pipe replacement were not lower than those of children who lived in housing with a complete lead service line.
Water from systems that serve <25 persons and water from private drinking water wells is not regulated under the Safe Drinking Water Act, so LCR does not apply to these water sources, and they are not routinely tested for lead. Approximately 40–45 million people in the United States drink water that is not subject to the LCR regulations (64). The number of homes supplied by private wells or sources that serve <25 persons that have leaded plumbing, fixtures, or solder is unknown, as is the number of such homes that would benefit from appropriate corrosion control or water filtration at the point of use.
Since 1991, when LCR was finalized and enforced, tap water lead levels have substantially decreased (65). However, conditions still exist that could allow children to be exposed to water lead levels ≥15 ppb. Drinking water from systems with lead service lines that do not have optimized corrosion control might not be in compliance with LCR, which can result in this level of lead exposure. A system with lead service lines that is in compliance with LCR can still expose children to lead levels ≥15 ppb because LCR permits ≤10% of sampled homes to exceed the action level of 15 ppb. Optimized corrosion control also might not completely prevent lead in plumbing materials from dissolving into drinking water. Persons who drink water from these sources might be exposed to lead levels >15 ppb.
Conclusion
Although EPA has the primary responsibility for ensuring the safety of drinking water, state and local childhood lead poisoning prevention programs are important partners in ensuring that the public is protected from lead exposure. These programs promote blood lead screening, conduct blood lead surveillance, provide clinician and public education and outreach, and provide case management for children with elevated BLLs. Because children with elevated BLLs might be exposed to many sources of lead, all sources of lead should be considered when their homes are inspected. Childhood lead poisoning prevention programs can obtain data on lead in public drinking water systems from the water suppliers (66). Drinking water in older housing should be tested as a source of lead exposure when the local drinking water system is not in compliance with LCR or when another source of lead exposure cannot be identified for children with high BLLs (67).
Data are not available to address certain issues regarding lead in drinking water. The effect of water lead levels on BLLs of children and other populations at risk for adverse effects is difficult to measure. Current water sampling protocols were designed to assess the adequacy of water treatment, not the level of human exposure to lead. Important fluctuations in water lead levels might be missed because of limitations inherent in sampling protocols developed for regulatory purposes. Future research efforts could validate the accuracy of sampling protocols for identifying fluctuations of lead concentrations, predicting exposure to lead at the individual level, and determining how best to measure lead concentrations in multifamily versus single-family homes, child-care centers, and school settings. As new alternatives to lead in plumbing are developed, they should be evaluated before being marketed. More research, surveillance, and intervention studies also are needed to determine the most effective ways to reduce the lead concentration of drinking water to meet the goal of eliminating high BLLs in children.
Childhood lead poisoning prevention programs routinely provide information on practices that minimize exposure to lead in water. This information can be updated with additional materials promoting lead-safe plumbing practices. Training curricula for lead exposure assessment protocols and case-management guidelines for children with elevated BLLs should incorporate lead-safe water and plumbing information. Appropriate risk communication materials for water customers that are accurate and reflect specific language and cultural factors might be beneficial.
Partial lead service line replacement has been associated with short-term increases in lead levels in drinking water (65) and has not been found to decrease risk for BLLs ≥5 µg/dL in children (62). These findings imply that the practice of partially replacing lead service lines as a method to comply with LCR should be reconsidered. One alternative is full replacement of lead service lines, regardless of whether the lead service line is owned by the water authority or the property owner. Lower BLLs could be achieved if plumbing components contained the lowest possible levels of lead and monitoring and enforcement activities were effective. Finally, information about lead in plumbing components, often available in tax assessor data, could be incorporated into information routinely provided to homebuyers or renters before they make the decision to buy or rent a property.
Since 1970, considerable reductions in lead concentrations have occurred in air, tap water, food, dust, and soil, which significantly reduced the BLLs of children throughout the United States. However, children are still being exposed to lead, and no safe blood lead threshold for children has been identified.
All sources of lead in the environments of children should be controlled or eliminated (2). For the many children living in housing built before 1978, lead sources include lead paint hazards as well as lead in plumbing components and fixtures. Children can still be exposed to tap water with lead levels of ≥15 ppb if they live in older homes that are more likely to have lead water pipes or fixtures. To prevent lead exposure from tap water, persons involved with childhood lead poisoning prevention programs should be familiar with communities having buildings at high risk for lead and monitor whether local water providers are in compliance with LCR (66). EPA is currently reviewing LCR, which provides an opportunity for continued collaboration between EPA and CDC that focuses on reducing the public health consequences of exposure to lead in water.
References
- Agency for Toxic Substances and Disease Registry. Toxicological profile for lead. Atlanta, GA: US Department of Health and Human Services, Agency for Toxic Substances and Disease Registry; 2007. Available at http://www.atsdr.cdc.gov/toxprofiles/tp13.pdf . Accessed June 5, 2012.
- CDC. Preventing lead poisoning in young children. Atlanta, GA: CDC; 2005. Available at http://www.cdc.gov/nceh/lead/publications/prevleadpoisoning.pdf . Accessed June 5, 2012.
- Advisory Committee on Childhood Lead Poisoning Prevention (ACCLPP). Low level lead exposure harms children: a renewed call for primary prevention. Atlanta, GA: US Department of Health and Human Services, CDC, ACCLPP; 2012. Available at http://www.cdc.gov/nceh/lead/ACCLPP/Final_Document_010412.pdf . Accessed July 2, 2012.
- Davidson CI, Rabinowitz M. 1992. Lead in the environment: from sources to human receptors [Chapter 4]. In: Needleman H, ed. Human lead exposure. Boca Raton, FL: CRC Press; 1992.
- Flegal AR, Smith DR. Lead levels in preindustrial humans. NEJM 1992;326:1293–4.
- Jones R, Homa D, Meyer P, et al. Trends in blood lead levels and blood lead testing among U.S. children aged 1 to 5 years: 1998–2004. Pediatrics 2009;123:e376–85
- Byers RK, Lord EE. Late effects of lead poisoning on mental development. Am J Dis Child 1943;66:471–94. Available at http://www.rachel.org/files/document/Late_Effects_of_Lead_Poisoning_on_Mental_devel.pdf Accessed June 5, 2012.
- Canfield RL, Henderson CR Jr, Cory-Slechta DA, Cox C, Jusko TA, Lanphear BP. Intellectual impairment in children with blood lead concentrations below 10 µg per deciliter. N Engl J Med 2003;348:1517–26.
- Lanphear BP, Hornung R, Khoury J, et al. Low-level environmental lead exposure and children’s intellectual function: an international pooled analysis. Environ Health Perspect 2005;113:894–9.
- Téllez-Rojo MM, Bellinger DC, Arroyo-Quiroz C, et al. Longitudinal associations between blood lead concentrations lower than 10 µg/dL and neurobehavioral development in environmentally exposed children in Mexico City. Pediatrics 2006;118:e323–30.
- US Environmental Protection Agency. Review of the national ambient air quality standards for lead: exposure analysis methodology and validation. Washington, DC: Air Quality Management Division, Office of Air Quality Planning and Standards; 1989. Available at http://www.epa.gov/ttnnaaqs/standards/pb/data/rnaaqsl_eamv.pdf . Accessed June 5, 2012.
- Bellinger DC, Stiles KM, Needleman HL. Low-level lead exposure, intelligence and academic achievement: a long-term follow-up study. Pediatrics 1992;90:855–61.
- Fergusson DM, Horwood LJ, Lynskey MT. Early dentine lead levels and educational outcomes at 18 years. J Child Psychol Psychiatry 1997;38:471–8.
- Ris MD, Dietrich KN, Succop PA, Berger OG, Bornschein RL. Early exposure to lead and neuropsychological outcome in adolescence. J Int Neuropsychol Soc 2004;10:261–70.
- Tong S, Baghurst PA, Sawyer MG, Burns J, McMichael AJ. Declining blood lead levels and changes in cognitive function during childhood: the Port Pirie Cohort Study. JAMA 1998;280:1915–9.
- Wasserman GA, Liu X, Popovac D, et al. The Yugoslavia Prospective Lead Study: contributions of prenatal and postnatal lead exposure to early intelligence. Neurotoxicol Teratol 2000;22:811–8.
- Klaassen C, ed. Casarett and Doull’s toxicology: the basic science of poison. 7th ed. New York City, NY: McGraw Hill; 2008.
- Goyer RA. Lead toxicity: from overt to subclinical to subtle health effect. Environ Health Perspect 1990;86:177–81.
- Nawrot TS, Thijs L, Den Hond EM, Roels Ha, Staessen JA. An epidemiological re-appraisal of the association between blood pressure and blood lead: a meta-analysis. J Hum Hypertens 2002;16:123–31.
- Menke A, Muntner P, Batuman V, Silbergeld EK, Guallar E. Blood lead below 0.48 µmol/l (10 µg/dl) and mortality among US adults. Circulation 2006;114:1388–94.
- Bellinger DC. Teratogen update: lead and pregnancy. Birth Defects Res A Clin Mol Teratol 2005;73:409–20.
- CDC. Guidelines for the identification and management of lead exposure in pregnant and lactating women. Atlanta, GA: CDC; 2010. http://www.cdc.gov/nceh/lead/publications/leadandpregnancy2010.pdf . Accessed June 5, 2012.
- Telisman S, Cvitkovic P, Jurasovic J, Pizent A, Gavella M, Rocic B. Semen quality and reproductive endocrine function in relation to biomarkers of lead, cadmium, zinc and copper in men. Environmental Health Perspective 2000;108:45–53.
- World Health Organization. Global health risks: mortality and burden of diseases attributable to selected major risks. Geneva, Switzerland: World Health Organization; 2009. Available at http://www.who.int/healthinfo/global_burden_disease/GlobalHealthRisks_report_full.pdf . Accessed June 5, 2012.
- Pruss-Ustun A, Vickers C, Haefliger P, Berollini T. Knowns and unknowns on burden of disease due to chemicals: a systematic review. Environ Health 2011;10:9.
- Levin R. Reducing lead in drinking water: a benefit analysis. Washington, DC: US Environmental Protection Agency; 1986. Report no. EPA 230-09-86-019. Available at http://yosemite.epa.gov/ee/epa/eerm.nsf/vwGA/491BF1DB78AABA2E8525651B006EFC06. Accessed June 5, 2012.
- Grosse S, Matte T, Schwartz J, Jackson R. Economic gains resulting from resulting from children’s exposure to lead in the United States. Environ Health Perspect 2002;110:563–9.
- Gould E. Childhood lead poisoning: conservative estimates of the social and economic benefits of lead hazard control. Environmental Health Perspect 2009;117:1162–7
- US Environmental Protection Agency. America’s children and the environment: measure B1: lead in the blood of children. Washington, DC: US Environmental Protection Agency; 2010. Available at http://www.epa.gov/ace/body_burdens/b1-graph.html. Accessed June 5, 2012.
- Mahaffey KR, Annest JL, Roberts J, Murphy MS. National estimates of blood lead levels: United States (1976–1980). N Engl J Med 1982;307:573–9.
- Klitzman S, Sharma A, Nicaj L, Vitkevich R, Leighton J. Lead poisoning among pregnant women in New York City: risk factors and screening practices. J Urban Health 2002;79:225–37.
- CDC. Blood lead levels in the United States, 1999–2002. MMWR 2005;54:513–6.
- Tehranifar P, Leighton J, Auchincloss AH, et al. Immigration and risk of childhood lead poisoning: findings from a case control study of New York City children. Am J Public Health 2008;98:92–7.
- Graber N, Gabinskaya T, Forman J, Gertner M. Prenatal lead exposure in New York City immigrant communities [Poster]. In: Pediatric Academic Societies (PAS) 2006 Annual Meeting; 2006; San Francisco, CA.
- Levin R, Brown MJ, Kashtock ME, et al. U.S. children’s lead exposures, 2008: implications for prevention. Environ Health Perspect 2008;116:1285–93.
- US Environmental Protection Agency. Air quality criteria for lead. Vol. 1 of 2. Section 3.1.2. Research Triangle Park, NC: US Environmental Protection Agency, Office of Research and Development, National Center for Environmental Assessment—RTP Division; 2006. Available at http://cfpub.epa.gov/ncea/cfm/recordisplay.cfm?deid=158823. Accessed July 2, 2012.
- Arizona Department of Health Services. Arizona’s children and the environment: a summary of the primary environmental health factors affecting Arizona’s children. Phoenix, AZ: Arizona Department of Health Services; 2003. Available at http://www.azdhs.gov/phs/oeh/pdf/gov_chldrn_hlth_rpt.pdf . Accessed June 5, 2012.
- Bernard SM, McGeehin MA. Prevalence of blood lead levels ≥5 µg/dL among U.S. children 1 to 5 years of age and socioeconomic and demographic factors associated with blood of lead levels 5 to 10 µg/dL, Third National Health and Nutrition Examination Survey, 1988–1994. Pediatrics 2003;112:1308–13.
- Chin D, Karalekas PCJ. Lead product use survey of public water supply distribution systems throughout the United States. In: Proceedings of Plumbing Materials and Drinking Water Quality Seminar; May 16-17, 1984; Cincinnati, Ohio. EPA 600/9-85-007:110–23. Washington, DC: US Environmental Protection Agency; 1985.
- Cosgrove E, Brown MJ, Madigan P, McNulty P, Okonski L, Schmidt J. Childhood lead poisoning: case study traces source to drinking water. J Environ Health 1989;52:346–9.
- Shannon M, Graef JW. Lead intoxication from lead-contaminated water used to reconstitute infant formula. Clin Pediatr 1989;28:380–2.
- Lanphear BP, Burgoon DA, Rust SW, Eberly S, Galke W. Environmental exposures to lead and urban children’s blood lead levels. Environ Res 1998;76:120–30.
- CDC. Blood lead levels in residents of homes with elevated lead in tap water—District of Columbia, 2004. MMWR 2004;53:268–70.
- Miranda ML, Kim D, Jull AP, Paul CJ, Overstreet Galeano MA. Changes in blood lead levels associated with use of chloramines in water treatment systems. Environ Health Perspect 2007;115:221–5.
- Amtsblatt des Königlichen Württembergischen Ministeriums des Innern [German]. Vol. 7. Stuttgart, Germany: Ministeriums des Innern; 1878:101–6.
- Karalekas PC, Ryan CR, Taylor FB. Control of lead, copper and iron pipe corrosion in Boston. J Am Water Works Assoc 1983:75:92–6.
- Donaldson W. The action of water on service pipes. J Am Water Works Assoc 1924;11:694.
- American Water Works Association; Weston RF. Lead service line replacement: a benefit to cost analysis. Denver, CO: American Water Works Association; 1990.
- Moore MR, Meredith PA, Campbell BC, Goldberg A, Pocock SJ. Contribution of lead in drinking water to blood-lead. Lancet 1977;2:661–2.
- Elwood PC, St Leger AS. Dependence of blood-lead on domestic water lead. Lancet 1976;1:1295.
- Schock MR. Causes of temporal variability of lead in domestic plumbing systems. Environ Monit Assess 1990;15:59–82.
- Lacey RF, Moore MR, Richards WN. Lead in water, infant diet and blood: the Glasgow duplicate diet study. Sci Tot Environ 1985;41:235–57.
- Worth D, Matranga A, Lieberman M, et al. Lead in drinking water: the contribution of household tap water to blood lead levels. In: Environmental lead. New York City, New York: Academic Press; 1981.
- Thomas HF, Elwood PC, Welsby E, St Leger AS. Relationship of blood lead in women and children to domestic water lead. Nature 1979;282:712–3. 2011.
- Pocock SJ, Shaper AG, Walker M, Wale CJ, Clayton B. Effects of tap water lead, water hardness, alcohol, and cigarettes on blood lead concentrations. Epidemiol Comm Health 1983;37:1–7.
- Watt GCM, Britton A, Gilmour WH, et al. Is lead in tap water still a public health problem? an observational study in Glasgow. BMJ 1996;313:979–81.
- Lanphear BP, Burgoon DA, Rust SW, Eberly S, Galke W. Environmental exposures to lead and urban children’s blood lead levels. Environ Res 1998;76:120–30.
- Lanphear BP, Matte TD, Rogers J, et al. The contribution of lead-contaminated house dust and residential soil to children’s blood lead levels: a pooled analysis of 12 epidemiologic studies. Env Res 1998;79:51–68.
- Tiemann M. Lead in drinking water: Washington DC, issues and broader regulatory implications. Washington, DC: Library of Congress, Congressional Research Service; 2004. Report no. RS 21831. Available at http://www.cnie.org/NLE/CRSreports/05jan/RS21831.pdf . Accessed June 5, 2012.
- Schock MR, Lytle DA. Internal corrosion and deposition control [Chapter 20]. In: Waterquality and treatment: a handbook of community water supplies. 6th ed. New York City, NY: McGraw-Hill; 2010.
- US Environmental Protection Agency. Elevated lead in D.C. drinking water—a study of potential causative events, final summary report. Washington, DC: US Environmental Protection Agency; 2007. Available at http://water.epa.gov/lawsregs/rulesregs/sdwa/lcr/upload/2007_09_12_lcrmr_pdfs_report_lcmr_elevatedleadindc_final.pdf . Accessed June 5, 2012.
- Brown MJ, Raymond J, Homa D, Kennedy C, Sinks T. Association between children’s blood lead service lines, and water disinfection, Washington, DC, 1998–2006. Environ Res 2012;111:67–74.
- Edwards M, Triantafyllidou S, Best D. 2009. Elevated blood lead in young children due to lead-contaminated drinking water: Washington, DC, 2001-2004. Environ Sci Technol 43:1618-23.
- US Department of Health and Human Services. The Surgeon General’s call to action to promote healthy homes. Washington, DC: US Department of Health and Human Services, Office of the Surgeon General; 2009. Available at http://www.surgeongeneral.gov/topics/healthyhomes/calltoactiontopromotehealthyhomes.pdf . Accessed June 5, 2012.
- AWWARF-TZW. Internal corrosion of water distribution systems. 2nd ed. American Water Works Association Research Foundation/DVGW-TZW: Denver, CO; 1996.
- Brown MJ. Important update: Washington DC blood lead level tests [Letter]. Atlanta, GA: March 20, 2010. Available at http://www.cdc.gov/nceh/lead/blood_levels.htm. Accessed June 5, 2012.
- CDC. Managing elevated blood lead levels among young children: recommendations from the Advisory Committee on Childhood Lead Poisoning Prevention. Atlanta, GA: CDC; 2002. Available at http://www.cdc.gov/nceh/lead/CaseManagement/caseManage_main.htm. Accessed June 5, 2012.
FIGURE. Timeline of lead poisoning prevention policies and blood lead levels in children aged 1–5 years, by year — National Health and Nutrition Examination Survey, United States, 1971–2008
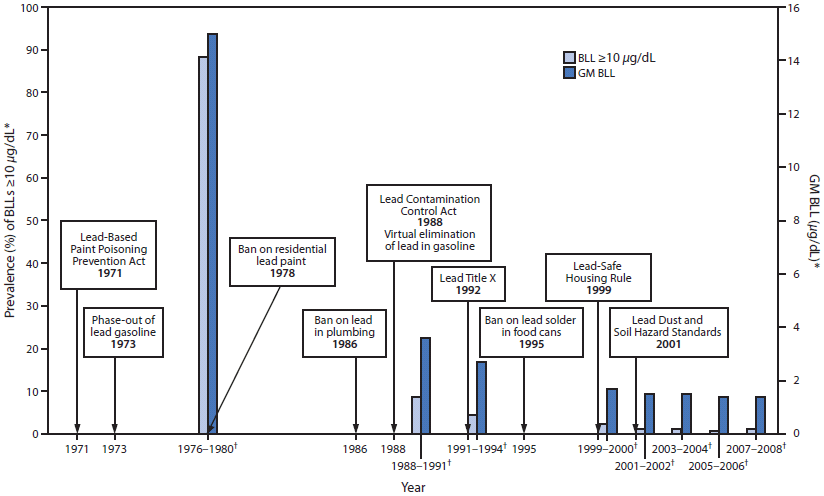
Abbreviations: BLL = blood lead level; GM = geometric mean; NHANES = National Health and Nutrition Examination Survey.
Sources: Mahaffey KR, Annest JL, Roberts J, Murphy MS. National estimates of blood lead levels: United States (1976–1980). N Engl J Med 1982;307:573–9. Jones R, Homa D, Meyer P, et al. Trends in blood lead levels and blood lead testing among U.S. children aged 1 to 5 years: 1998–2004. Pediatrics 2009;123:e376-85. National Health and Nutrition Examination Survey, 2000–2008. Available at http://www.cdc.gov/nchs/nhanes.htm. Accessed July 17, 2012.
* National estimates for GM BLLs and prevalence of BLLs ≥10 µg/dL, by NHANES survey period and sample size of children aged 1–5 years: 1976–1980: N = 2,372; 1988–1991: N = 2,232; 1991–1994: N = 2,392; 1999–2000: N = 723; 2001–2002: N = 898; 2003–2004: N = 911; 2005–2006: N = 968; 2007–2008: N = 817.
† NHANES survey period.
Alternate Text: This figure shows a timeline of lead poisoning prevention policies combined with a graph of blood lead levels (BLLs) in children aged 1–5 years, by year, during 1971–2008. BLL data are from the National Health and Nutrition Examination Survey. The lead policies include the Lead-Based Paint Poisoning Prevention Act in 1971 , the phase-out of lead gasoline in 1973, the ban on residential lead paint in 1978, the ban on lead in plumbing in 1986, the Lead Contamination Control Act in 1988, which led to the virtual elimination of lead in gasoline, Lead Title X in 1992, the ban on lead solder in food cans in 1995, the Lead-Safe Housing Rule in 1999, and the Lead Dust and Soil Hazard Standards in 2001. The left y-axis show the geometric mean (GM) BLLs. GM BLLs decreased from 15 µg/dL during 1976–1980 to 3.6 µg/dL during 1988–1991 to 1.4 µg/dL during 2007–2008. The prevalence of BLLs ≥10 µg/dL decreased from 88.2% during 1976–1980 to 8.6% during 1988–1991 to 1.2% during 2007–2008.